Quality Control for RNA Therapeutics: Meeting a Growing Need
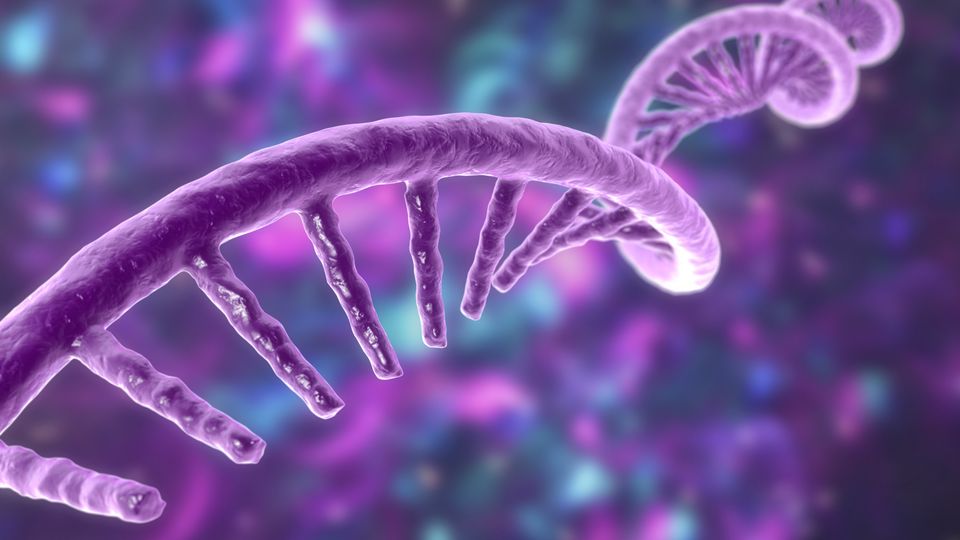
Complete the form below to unlock access to ALL audio articles.
RNA therapeutics played a pivotal role in suppressing the global prevalence of COVID-19, thanks to the highly efficacious Pfizer/BioNTech and Moderna mRNA vaccines. While the development of RNA therapeutics was accelerated by the race to develop a COVID-19 vaccine, the associated influx of scientific breakthroughs in the RNA therapeutic space highlighted this technology's immense potential to treat various diseases.
Indeed, 21 RNA therapeutics spanning various therapeutic areas are currently approved or in late-stage trials. Some disease indications that current late-stage RNA therapeutics address include atherosclerosis, cytomegalovirus retinitis, coronary artery disease, Duchenne Muscular Dystrophy (DMD), orphan genetic diseases and many more. This article will cover the available RNA therapies and provide an overview of their applications beyond vaccinating against COVID-19. Moreover, the critical need for effective quality control when developing and manufacturing these therapeutics will be highlighted, along with the key techniques that ensure their safe and effective delivery.
The wide world of RNA therapeutics
RNA therapeutics are relatively simple and inexpensive to design and manufacture, making them a cost-effective option compared to other types of precision medicine. Additionally, RNA can be manipulated to target any coding or non-coding region of the genome. As a result, researchers can design RNA therapeutics to treat a broader range of rare and common diseases unreachable by small molecule drugs and therapeutic antibodies since these can only target 0.05% of proteins encoded by the human genome. RNA therapeutics can be designed in various forms depending on their purpose. Major classes of RNA therapeutics include (1) mRNA vaccines, (2) antisense oligonucleotides (ASOs), (3) RNA interference (RNAi), (4) RNA aptamers and (5) CRISPR/nuclease systems.
mRNA vaccines can train the immune system to attack any target, from a virus to a tumor, as exemplified by the successful COVID-19 mRNA vaccines and by Moderna and Merck's joint, clinical-stage cancer vaccine, respectively. On the other hand, ASOs are short, single-stranded oligonucleotides designed to alter target RNA and increase, reduce or repair aberrant protein expression underlying disease phenotypes. This approach has resulted in the approval of several drugs that correct splicing defects associated with muscular dystrophy, including some personalized treatments. Further, RNAi is an RNA therapeutic that uses short, interfering RNAs (siRNAs) or microRNAs (miRNAs) to inhibit gene expression to treat, for example, the neurological disorder hTTR with polyneuropathy. RNA aptamers are short single-stranded nucleic acids forming secondary and tertiary structures that interact with and inhibit disease-associated enzymes or molecules. RNA aptamers are unique from other RNA therapeutics because they can target disease at the protein level to block unwanted protein activity to treat diseases such as macular degeneration. Finally, CRISPR nuclease systems employ DNA-targeting, sequence-specific guide RNAs to direct nucleases to permanently edit the genome, as exemplified by the reversion of mutations that cause the blood disorder ß-thalassemia and sickle cell anemia.
Cells have natural barriers that stop naked therapeutic RNAs from reaching their target tissues, so these therapies require a delivery system to penetrate cells. Developers may use various chemical modifications or nanocarriers to ensure that RNA gets where it needs to be in sufficient quantities.
While RNA therapeutics represent an exciting and expansive area of precision medicine, ultrasensitive techniques are needed to assess and validate their quality, safety and efficacy.
Quality control requirements in RNA therapy manufacturing
Compared to small molecule pharmaceuticals and therapeutic antibodies, RNA therapeutics require a roster of quality control tests centered around precise nucleic acid quantification. These tests must provide:
- Quantification of the therapeutic RNA to ensure accurate dosing
- Quantification of degraded or contaminant nucleic acids to ensure safety
Droplet Digital PCR (ddPCR) can provide absolute quantification of nucleic acids with the exceptionally high precision and accuracy necessary to ensure a high-quality product. ddPCR technology has already proven valuable in evaluating necessary parameters with high precision and accuracy during COVID-19 vaccine development and beyond.
Running a ddPCR assay starts with partitioning a sample into tens of thousands of oil droplets suspended in water, each carrying one nucleic acid strand on average. Next, a standard PCR reaction occurs within each droplet, amplifying the target sequence with primers containing a fluorescent tag. At the end of the reaction, a droplet reader counts the positive (fluorescent) droplets and the negative (non-fluorescent) droplets. Finally, Poisson statistics are used to retrospectively determine the absolute quantity of target nucleic acids in the original sample.
While many researchers use reverse transcriptase-quantitative PCR (RT-qPCR) to make these measurements, this technique is less sensitive than ddPCR technology, particularly when evaluating small quantities of a target sequence or when large amounts of endogenous RNA mask the target RNA sequence. Moreover, the standard readout for RT-qPCR is relative quantification, and a manually-diluted standard curve is required for absolute quantification. This extra step introduces the possibility of human error, reducing assay sensitivity.
Using ddPCR technology to optimize RNA therapeutic quality
In practice, researchers and manufacturers may use ddPCR technology and RT-qPCR assays in tandem. Still, when developing a new therapy, they should be conscientious of the limits of their RT-qPCR assays and leverage ddPCR technology when applicable. Indeed, many researchers are already turning to ddPCR technology to ensure their products are of the highest quality.
In one study, the scientists behind the Pfizer/BioNTech COVID-19 mRNA vaccine used ddPCR technology to characterize an essential element of product integrity: the poly(A) tail. The poly(A) tail on mRNA molecules that comprise mRNA vaccines ensures that the RNA will be translated and protected from degradation. If large quantities of the product degrade, it can reduce vaccine efficacy. However, using ddPCR technology, the authors established that a sufficient amount – 85 percent – of their RNA product retained the poly(A) tail.
In another study, researchers established that ddPCR technology was the ideal method for evaluating an ASO-based therapeutic for treating DMD. A lack of functional dystrophin causes lethality in DMD patients, but therapies that induce exon skipping adjacent to DMD-causing mutations can restore dystrophin expression. The researchers compared PCR, ddPCR, nested PCR and RT-qPCR technologies to unify the evaluation of these new exon-skipping therapies. They found that ddPCR technology returned the most precise, quantitative, reproducible results for quantifying exon skipping efficacy.
In a third study, researchers developing a treatment for ß-thalassemia reported a CRISPR/Cas9-based approach to replace the impaired α-globulin gene with a functional ß-globulin gene. They used ddPCR technology to quantify the integration of the functional ß-globulin gene and to verify its insertion in the correct genomic location (at the α-globulin locus). Using ddPCR technology, they established that the ß-globulin gene integrated at a frequency of about 0.8 copies per cell with low-to-undetectable off-target integration, confirming the therapeutic gene dose of the treatment and establishing the groundwork for future clinical development.
The future of RNA therapeutics
The impressive quantitative power of ddPCR technology within the RNA therapeutic space illustrates its capacity to uphold quality, efficacy and safety standards during RNA therapeutic manufacturing for current and future applications.
The development of firm quality control measures and techniques for ensuring the safety and efficacy of RNA therapeutics stands to advance the field significantly. Given its unparalleled ability to directly quantify nucleic acids with high precision and accuracy, ddPCR technology will likely continue to set the standard for quality control for RNA therapeutics, enabling their development now and in the future. Furthermore, with ddPCR technology in their toolkit, developers can easily design and tailor RNA therapeutics, giving them an advantage in the pharmaceutical arena moving forward.
Optimizing methods to bring safe and effective RNA therapeutics to market will open the floodgates, setting developers up to tackle previously untreatable diseases, cure rare genetic disorders, and mobilize to mitigate future viral outbreaks like the COVID-19 pandemic.